Another fundamental question that fuels our research endeavors is the following: how can quantum coherence and correlations serve as tangible resources for quantum technologies? These are the very quantities that embody the delocalization of information in space. Is there a profound connection between delocalized quantum information and energy? We can focus on the irreversible processes and explore how quantum coherence and correlations can tangibly emerge as a source of power.
When two or more irreversible processes coexist in a system, they can couple with each other and generate crucial effects with practical applications, such as thermoelectricity, thermophoresis, and electrophoresis. Thermoelectricity, for instance, plays a significant role in increasing energy efficiency by converting waste or excessive heat into electricity and in solid-state refrigeration by operating in reverse. Although thermoelectric effects were discovered experimentally by Seebeck and Peltier between 1821 and 1834, the underlying theory remained immature until Lars Onsager published his famous reciprocal relations between heat and charge in 1931. Onsager based his theory on a 1981 paper by Lord Rayleigh, who focused for the first time on the entire dynamical process by which a gas of particles reaches a thermodynamic steady state. He captured the essential physics by developing a simplified collision model, which, in turn, allowed him to reveal the role of the environment’s initial conditions in heat conduction.
During his postdoctoral research at Koç University, Dr. Onur Pusuluk followed in the footsteps of Lord Rayleigh and Lars Onsager by systematically establishing quantum reciprocal relations between heat and quantum correlations, for which they introduced the term thermocoherent effect [J.4]. Our ongoing research activities in quantum thermodynamics focus on the applications of thermocoherence. Furthermore, we explore the thermocoherence within the perspective of quantum resource theories.
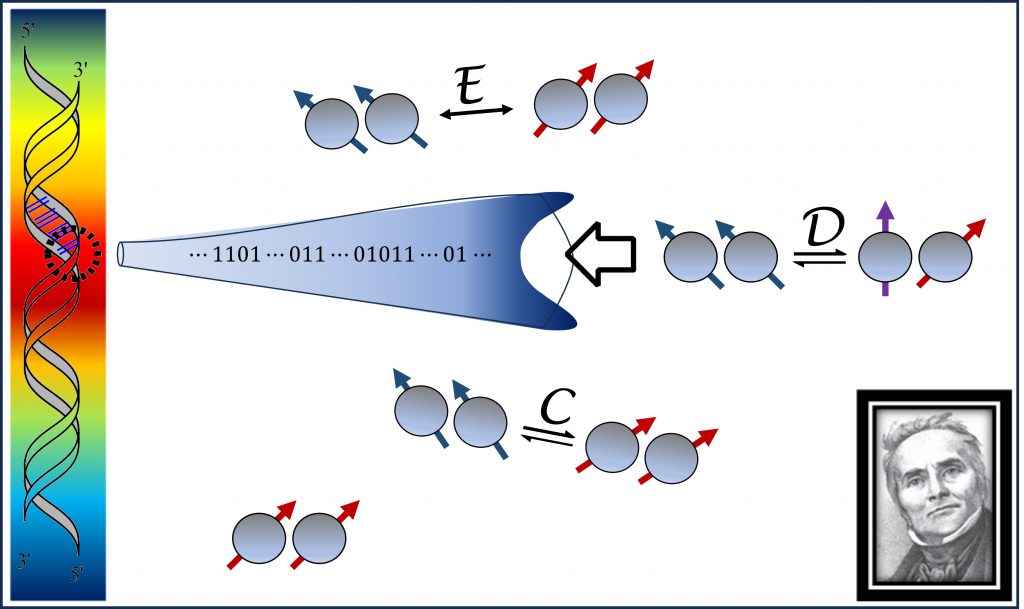
We can inject quantum correlations into a region to make compact non-thermal cooling or heating environments where injecting heat would be inefficient. This effect may pave the way for temperature manipulation within a single molecule and has wide-ranging ramifications for physics, biology, and chemistry. For example, Peltier devices control temperature-sensitive biochemical reactions such as DNA replication in the polymerase chain reaction (PCR) technology. We plan to develop thermocoherent cooling/heating devices to surpass the current state-of-the-art PCR techniques. Utilization of quantum coherence could allow for copying specific DNA sequences without requiring a heat-stable DNA polymerase enzyme, a necessary component of PCR machines.
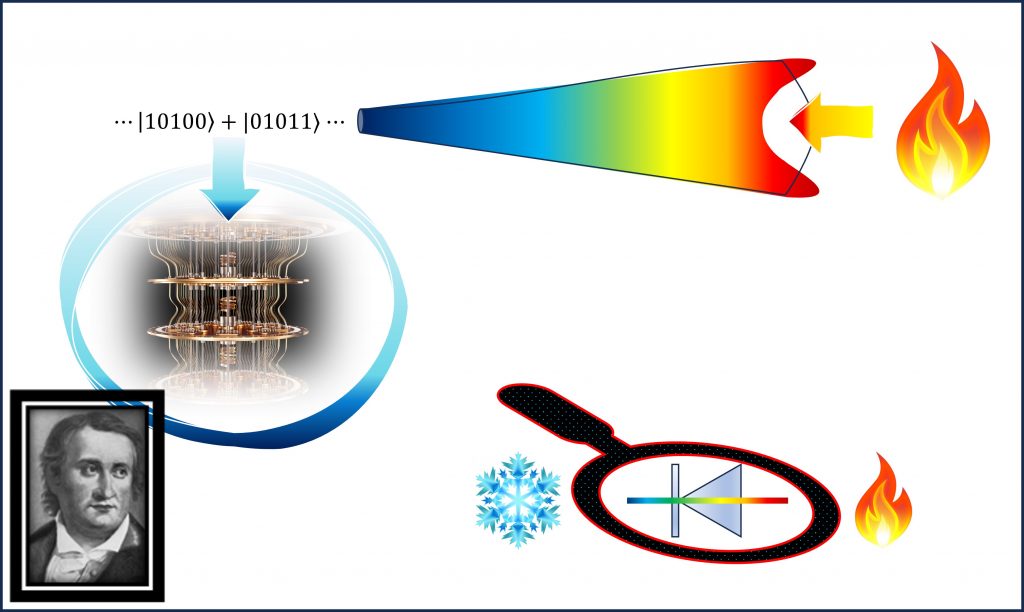
We can produce quantum correlations through a thermal gradient. Phononic devices, such as thermal diodes and transistors, can rectify heat flows on the micro and nanoscales, making them particularly useful in the thermocoherent preparation of qubits for information processing and thermodynamic tasks. In this regard, we investigate the possibility of designing quantum technologies based on phononics rather than electronics. Additionally, we explore the possibility of preserving quantum correlations in a reservoir by optimizing thermocoherent coefficients. We plan to use biochemical molecules or bioinspired artificial systems to design such dicoherent materials.
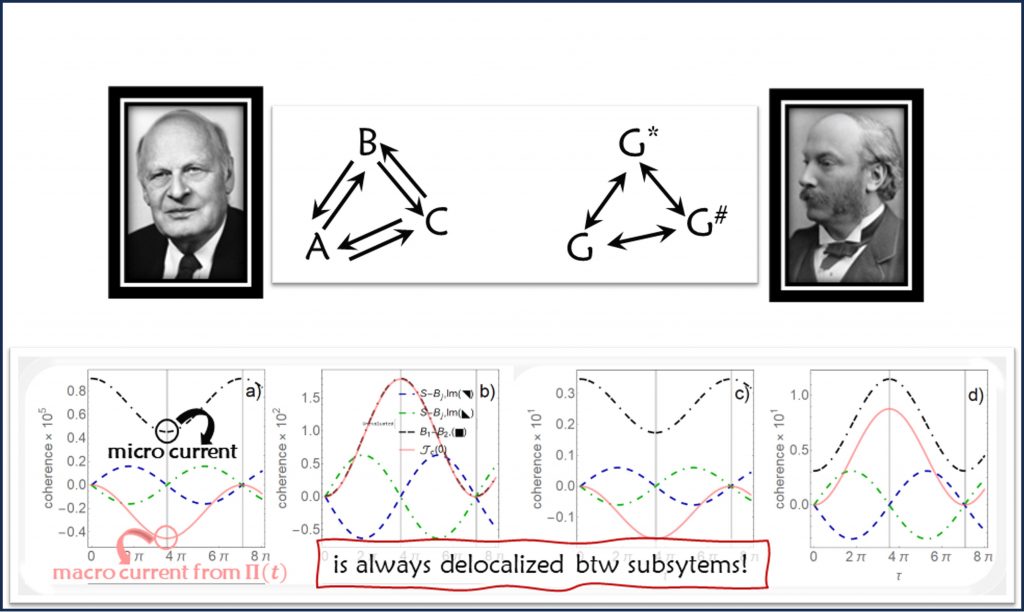
In his pioneering 1931 paper, Onsager investigated a chemical monomolecular triangle reaction A ↔ B ↔ C ↔ A to illustrate his reciprocity relations. Although this investigation was limited to currents for population fractions, a molecule can exist in the quantum coherent superposition of two electronic configurations. According to thermocoherent phenomena, quantum coherences generate new flows to or from the third configuration, which can coherently change the chemical equilibrium. We investigate various biochemical reactions, such as DNA replication, from the standpoint of thermocoherent effects.